Abstract
This manuscript describes the development of an extreme-range normobaric hypoxic environment (FO2 6.5%; PO2 31.4 kPa; simulated altitude 9300 m) utilising readily available commercial equipment and a low-cost portable hypoxia generator. The experimental configurations described validate the working hypothesis that reducing atmospheric oxygen partial pressure of air intake into a portable normobaric hypoxia generator will result in further oxygen depletion of Normobaric hypoxia (NH) air produced by the device. Within appropriately controlled conditions, creating extreme-range normobaric hypoxic environments may interest selected military personnel and high-altitude mountaineers for conducting training and research activities involving simulated rapid ascent exposures to extreme altitude.
Introduction
Normobaric hypoxia (NH) generators are typically used by endurance athletes as a method of obtaining adaptive training responses for marginal performance gains by exposure to oxygen-depleted environmental conditions. NH represents a low-cost and relatively convenient method of hypoxic stimulus training compared to natural environmental altitude exposure or the use of a hypobaric chamber. Athletic hypoxic adaptation can be conducted by either intermittent hypoxic training or chronic passive hypoxic exposure. Intermittent hypoxic training involves simultaneously subjecting the athlete to NH conditions while performing exercise. Chronic passive exposure is where the athlete is exposed to NH for extended durations throughout the day while at rest, typically by sleeping in a semi-closed altitude tent enclosure or purpose-designed chamber to simulate a ‘train low, sleep high’ conditioning cycle.
Portability, convenience and affordability have resulted in small-sized NH generators gaining popularity as a surrogate method of altitude acclimatisation for mountaineers and for conducting research on respiratory function at altitude. Commercially available portable NH generators are typically limited to generating hypoxic air with oxygen fraction (FO2) approximating 9%–9.5% with an estimated partial pressure of oxygen (PO2) of 44 kPa, simulating an altitude of 6700 m. While this exposure range is sufficient for the adaptation requirements of endurance athletes, obtaining more extreme simulated exposures may be of interest to selected military personnel and high-altitude mountaineers for conducting training and research activities involving higher altitudes.
This manuscript describes the development of an extreme-range normobaric hypoxia environment (FO2 6.5%; PO2 31.4 kPa; simulated altitude 9300 m) using readily available commercial equipment and a standard portable NH generator.
Materials and methods
Characteristics of JAY-10H hypoxic generator during normal operational use
JAY-10H (Longfian Scitech; Hebei, China) is a portable NH generator with characteristics typical for a personal use hypoxic training device (Figure 1). Technical parameters of the JAY-10H generator are listed in Table 1. The JAY-10H has two output gas outlets. The high-concentration oxygen gas outlet (FO2 93%) represents the waste by-product of NH gas production. A flow metre on the right of the front console can adjust the high-concentration oxygen flow rate. NH output volume is dependent on the NH gas concentration selected. NH output volumes up to 100 litres per minute are only obtained when less hypoxic air is being generated. When the most oxygen-depleted NH air output (FO2 9%) is generated, the production volume rate is substantially reduced.
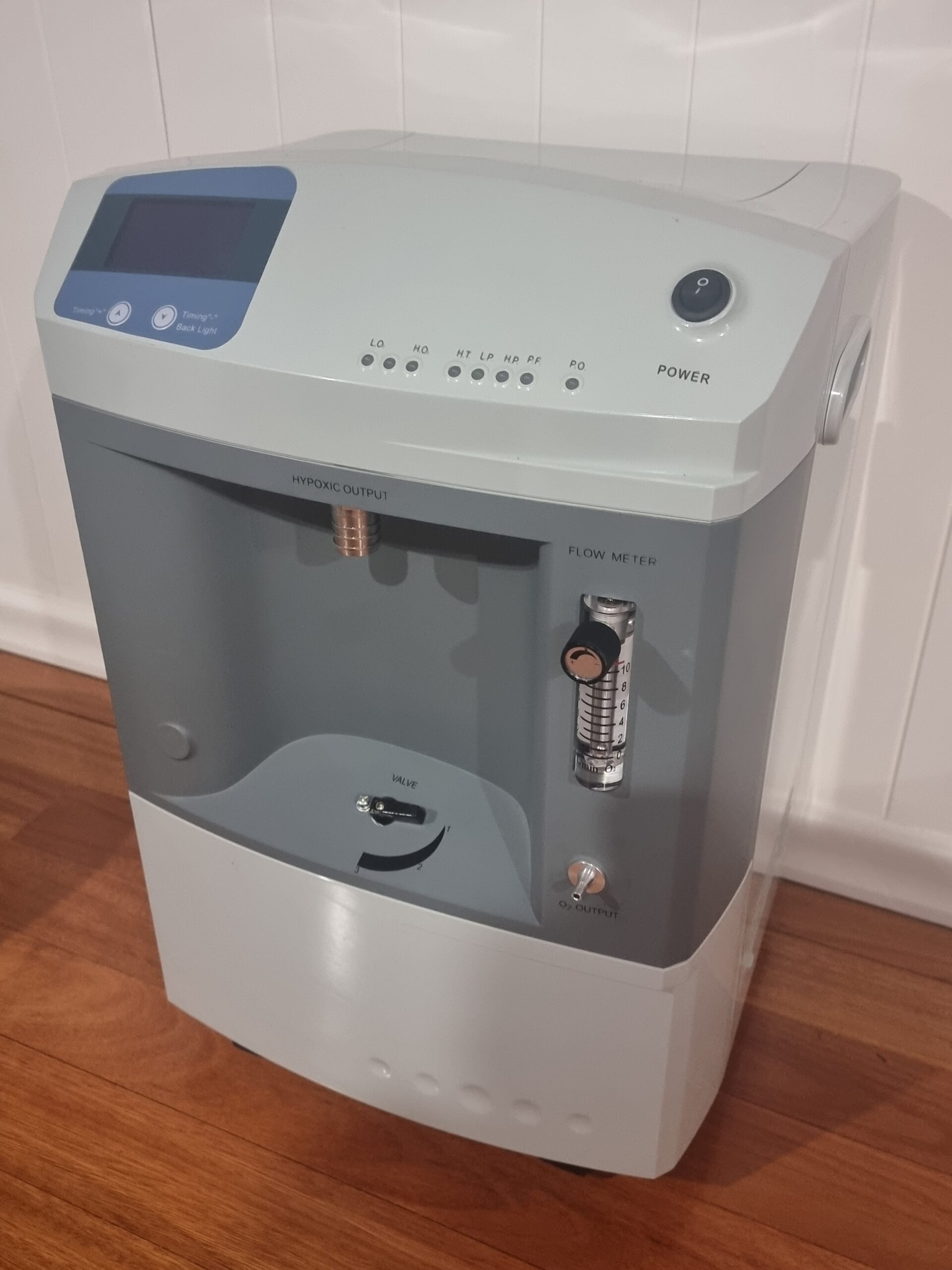
Figure 1: JAY-10H 100-litre hypoxic generator (Longfian Scitech Co. Ltd; Hebei, China).
Model | JAY-10H |
Weight | 26.7 kg |
Dimensions (cm) | 58 (height), 37 (width), 36 (depth) |
Maximum output flow | 100 Litres per minute (when FO2 20.9%) |
Minimum FO2 | 9% |
Minimum PO2 | 44 kPa |
Maximum simulated altitude | 6800 m |
Table 1: Technical parameters of the JAY-10H 100-litre hypoxic generator (Longfian Scitech Co. Ltd; Hebei, China).
The JAY-10H NH generator creates hypoxic air in a pulsatile waveform at a rate of 9 cycles per minute. The waveform is characterised by a short phase of high-flow rate NH air production followed by a longer duration phase of low flow rate NH air production. During the high-flow rate phase of the cycle, the NH air produced is of lower FO2 compared to the low flow rate phase. Due to the pulsatile NH output of the generator, a reservoir bag is commonly used to blend the oxygen-depleted gas to achieve greater consistency of user-inspired FO2.
Validation testing of JAY-10H hypoxic generator during normal operational use
Initial validation testing was conducted to confirm the working characteristics of the JAY-10H hypoxic generator in normal operational use. Validation testing and all subsequent experimentation were conducted in temperature-controlled normobaric normoxic conditions at sea level (25oC; 1012 kPa; FO2 20.9%; altitude <50 m). Environmental conditions were monitored using a Kestrel 4250 Racing Weather Tracker (Nielsen-Kellerman; Pennsylvania, USA). Ambient oxygen levels and NH oxygen concentration were monitored using a SmartSensor AR8100 Oxygen Meter (Smartsensor; Donguan, China).
For validation testing, NH air output was directly connected to a 1000-litre capacity reservoir bag (Qingdao Hinor Medical Industry Co. Ltd.; Shandong, China) to obtain a stable average output oxygen concentration with respect to the cyclic generator output (Figure 2; Figure 3). A 1000 litre reservoir bag was selected as prior pilot testing demonstrated a smaller volume 100 litre reservoir bag was insufficient for this purpose. Outflow from the reservoir bag was connected to tubing with a one-way valve at the end to prevent retrograde airflow into the system. Oxygen concentration within the output tubing was monitored by an oxygen meter (SmartSensor AR8100; Donguan, China) connected by a low-volume T piece. Using this method, a stable and reproducible average oxygen concentration from the generator could be determined under various conditions once the reservoir bag was completely inflated. Validation testing was conducted three times for each of three separate settings of the hypoxic flow regulator using unrestricted (high rate) waste oxygen flow or restricted waste oxygen flow (2 litres per minute). Complete deflation of the reservoir bag was conducted before each test. Altitude equivalency was calculated to the nearest 100m using the Baillie Lab Altitude Air Pressure Calculator (Roslin Institute; University of Edinburgh).1
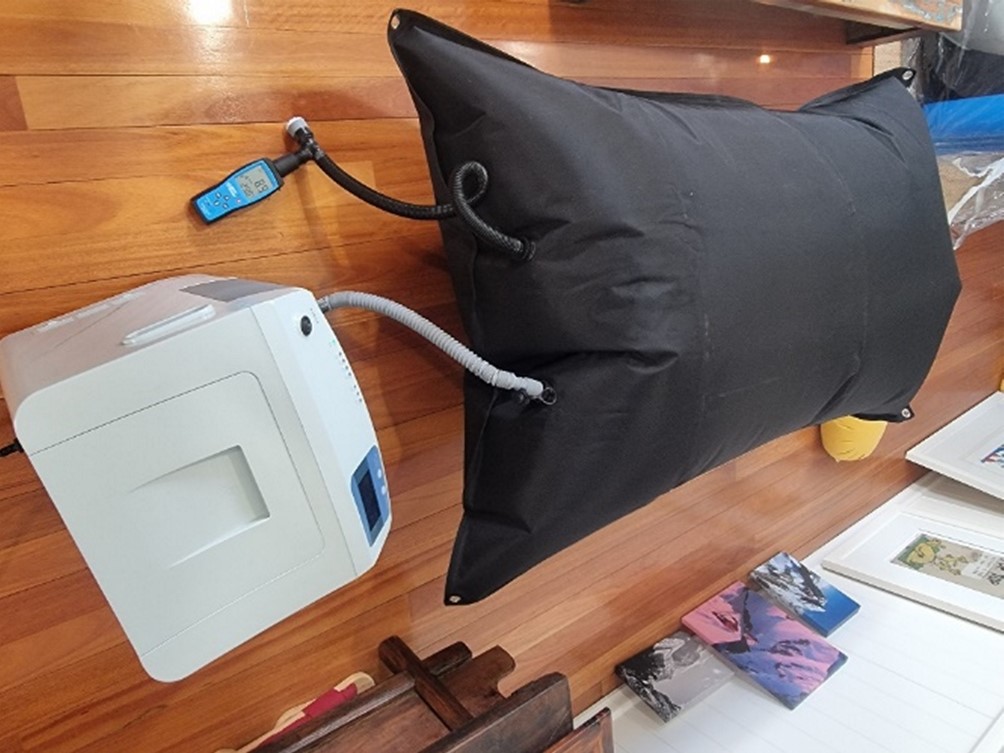
Figure 2 – JAY-10H validation testing configuration with inflated 1000-litre reservoir bag. Schematic configuration is demonstrated in Figure 3.
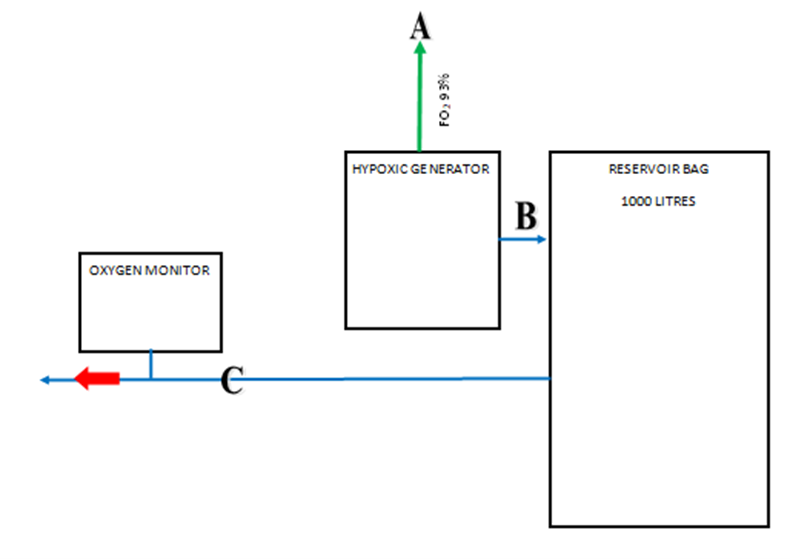
Figure 3: Schematic of JAY-10H hypoxic generator validation study. Green arrow (A): High oxygen concentration waste output. Blue: Normobaric hypoxic air output. Red arrow: One-way valve with directionality marked. B: Cyclic concentration hypoxic air output directly from the generator. C: Mixed hypoxic air from reservoir bag without cyclic variability of oxygen concentration.
Lowest (most oxygen depleted) FO2 readings were obtained using a combination of Position 1 of the hypoxic flow regulator together with unrestricted (high rate) oxygen regulator flow. This configuration obtained an average FO2 of 9.2% (standard deviation 0.3%), simulating an approximate hypobaric altitude of 6800 m (PO2 44.5 kPa).
The results of these validation tests demonstrated the JAY-10H hypoxic generator to be a consistently reliable device for creating depleted oxygen air, with the lowest and highest average recorded FO2 readings closely approximating those published by the manufacturer.2
After initial validation testing, the JAH-10H was connected to a standard 2080-litre altitude tent to assess the degree of oxygen depletion achieved during standard operational techniques for passive NH exposure conditioning (Figure 4). FO2 obtained within the altitude tent using this configuration was 11.4% (PO2 55.1 kPa; simulated altitude 5100 m). The difference between FO2 readings obtained from within the altitude tent (11.4%) compared to initial validation testing using the 1000-litre reservoir bag (9.2%) represents the effect of two-way air leakage from the altitude tent enclosure.
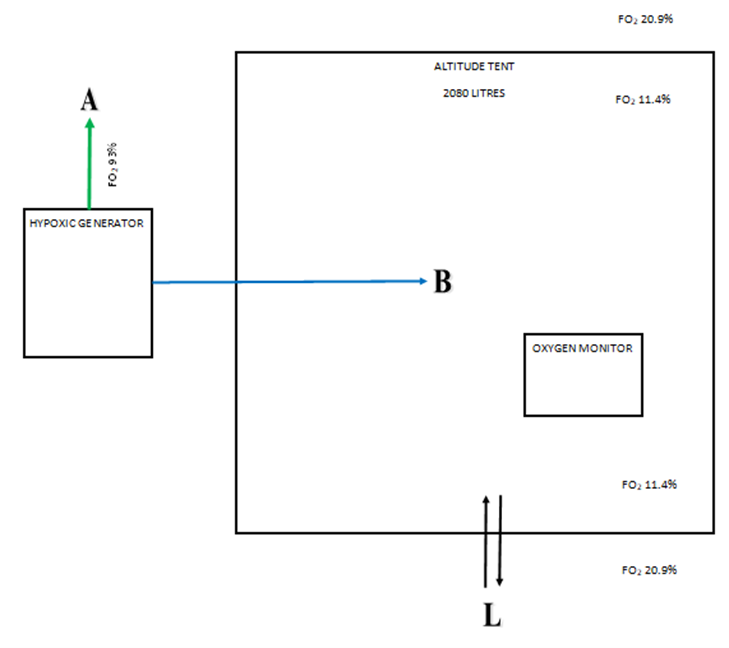
Figure 4: Schematic of system testing for standard operational use of the JAY-10H generator with a 2080-litre altitude tent used for resting subject hypoxic conditioning. A (green arrow): Highly oxygenated (FO2 93%) waste output from a hypoxic generator. B: Depleted oxygen generator output. L: Two-way leak rate between altitude tent and surrounding atmosphere.
System configurations for extreme-range normobaric hypoxic air generation
Performance and technical specifications of output from a portable NH generator assume employment of the device within normobaric normoxic conditions (101 kPa, FO2 20.9%). Manipulation of these baseline operating conditions will likely impact device performance and, therefore, NH output. The working hypothesis of the following experiments was that reducing ambient oxygen partial pressure of air intake into the JAY-10H generator would result in further depletion of NH air produced by the device.
Due to the incremental nature of how the test systems were developed, each configuration will be presented sequentially, including results and discussion relevant to each configuration.
Extreme-range NH test configuration 1
The first extreme-range NH experimental configuration involved placing the JAY-10H generator inside the 2080-litre altitude tent enclosure. Oxygen-rich generator waste gas was extracted from the system by routing the generator output tubing outside the tent enclosure. NH gas output was thus recirculated within the semi-closed system for subsequent reuptake by the generator (Figure 5). Altitude tents are not completely closed system as a degree of two-way gas leakage is expected, thus impacting the level of oxygen depletion obtained within the enclosure.
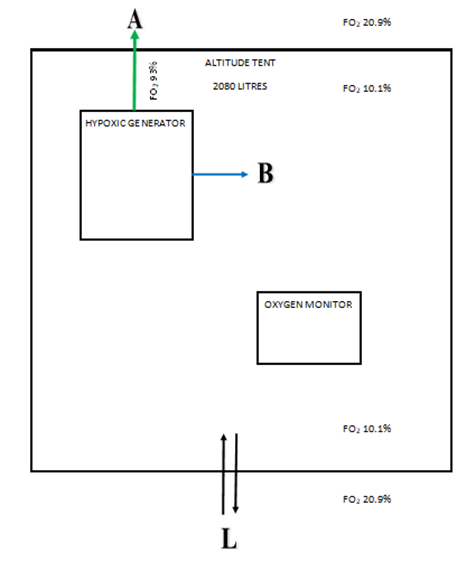
Figure 5: Extreme-range NH test configuration 1 schematic. A (green arrow): Highly oxygenated (FO2 93%) waste output from hypoxic generator removed from the system into atmosphere. B: Depleted oxygen output into altitude tent enclosure. L: Two-way leak rate between altitude tent and surrounding atmosphere.
Using this method, the JAY-10H generator created a steady-state FO2 of 10.1% within the tent enclosure (PO2 48.8 kPa; simulated altitude 6200 m). As the altitude tent was effectively acting as large-size reservoir bag, cyclic fluctuation of FO2 was not observed. Therefore, a test subject placed inside the altitude tent with the generator in this configuration would be exposed to a simulated altitude of 6200 m.
The environment created using this configuration was less oxygen depleted than obtained from a closed system 1000-litre reservoir bag with generator input of 20.9% oxygen during initial validation testing (FO2 9.2%). While test configuration 1 failed to achieve further oxygen depletion of NH output when compared to the more standard configuration (FO2 10.1% v 9.2%), the oxygen saturation obtained was, however, lower than compared to when the generator was connected to the altitude tent but located outside the enclosure (FO2 11.4%, Figure 4). These observations illustrate the impact of leakage into a semi-closed system on the levels of ambient oxygen saturation obtained within a tent enclosure. However, the results also demonstrate that reducing generator intake oxygen partial pressure does result in a further reduction in NH output, thus validating the initial hypothesis.
However, several practical limitations exist in using this configuration for conditioning and research. First, the oxygen depletion obtained is no more effective than what can be obtained using standard generator configurations. Furthermore, conditions for test subjects placed inside an altitude tent with a NH generator in operation would limit tolerance of this method due to excessive heat, noise and carbon dioxide accumulation.
Test configuration 1 did not provide a practical or effective method for generating extreme-range NH conditions. However, it did provide proof of concept for further development of extreme-range NH generation by subjecting the device to oxygen-depleted normobaric air intake.
Extreme-range NH test configuration 2
The second extreme-range NH configuration evaluated was an extension of the previous method where the JAY-10H generator was placed inside the 2080-litre altitude tent from which oxygen-rich waste gas was extracted. However, NH gas output was now connected to a split tubing system, each fitted with one-way valves (Figure 6). Using this configuration, human test subjects could be situated outside the altitude tent and breathe depleted NH air using a mask, thereby eliminating problems associated with heat, noise and carbon dioxide accumulation observed when subjects are situated inside the altitude tent. NH output from the generator was either directed to the subject for on-demand ventilation or diverted back into the altitude tent for recirculation and further oxygen depletion. Output FO2 was measured off the test subject gas line using a T piece.
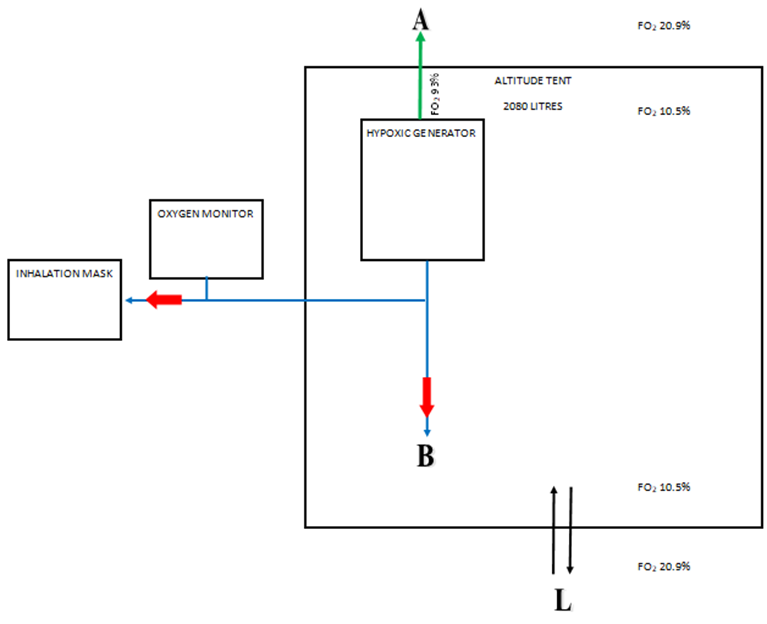
Figure 6: Extreme-range NH configuration 2 schematic. A (green arrow): Highly oxygenated (FO2 93%) waste output from hypoxic generator removed from the system into atmosphere. Blue arrows: Movement of depleted oxygen generator output. B: Recirculated NH output. L: Two-way leak rate between altitude tent and surrounding atmosphere. Green arrow: High-concentration waste oxygen output. Red arrows: One-way valves with directionality marked.
Due to the removal of the intervening reservoir between the JAY-10H generator and the subject gas line, this test configuration resulted in a cyclic NH gas output. Maximum and minimum oxygen percentages of the cyclic NH output are demonstrated in Table 2 and Figure 7. FO2 NH output decreased steadily over 2 hours from initiation of the experiment, after which a steady state of output was maintained with a cyclic minimum FO2 4.3% and a cyclic maximum FO2 7.5%.
TIME (Min) | MIN OUTPUT FO2 (%) | MAX OUTPUT FO2 (%) |
0 | 9.3 | |
5 | 8.9 | |
10 | 8.2 | |
15 | 8.1 | |
20 | 7.5 | |
25 | 6.9 | |
30 | 6.8 | |
35 | 6.5 | |
40 | 6.1 | |
45 | 6.0 | |
50 | 5.8 | |
55 | 5.6 | |
60 | 5.4 | |
65 | 5.2 | 8.8 |
70 | 5.1 | 8.9 |
75 | 5.0 | 8.6 |
80 | 4.8 | 8.5 |
85 | 4.8 | 8.6 |
90 | 4.8 | 8.1 |
95 | 4.6 | 7.9 |
100 | 4.5 | 7.9 |
105 | 4.4 | 7.9 |
110 | 4.4 | 7.7 |
115 | 4.3 | 7.6 |
120 | 4.3 | 7.5 |
Table 2: Generator cyclic FO2 output ranges in relation to time while operating in normobaric extreme hypoxia test configuration 2
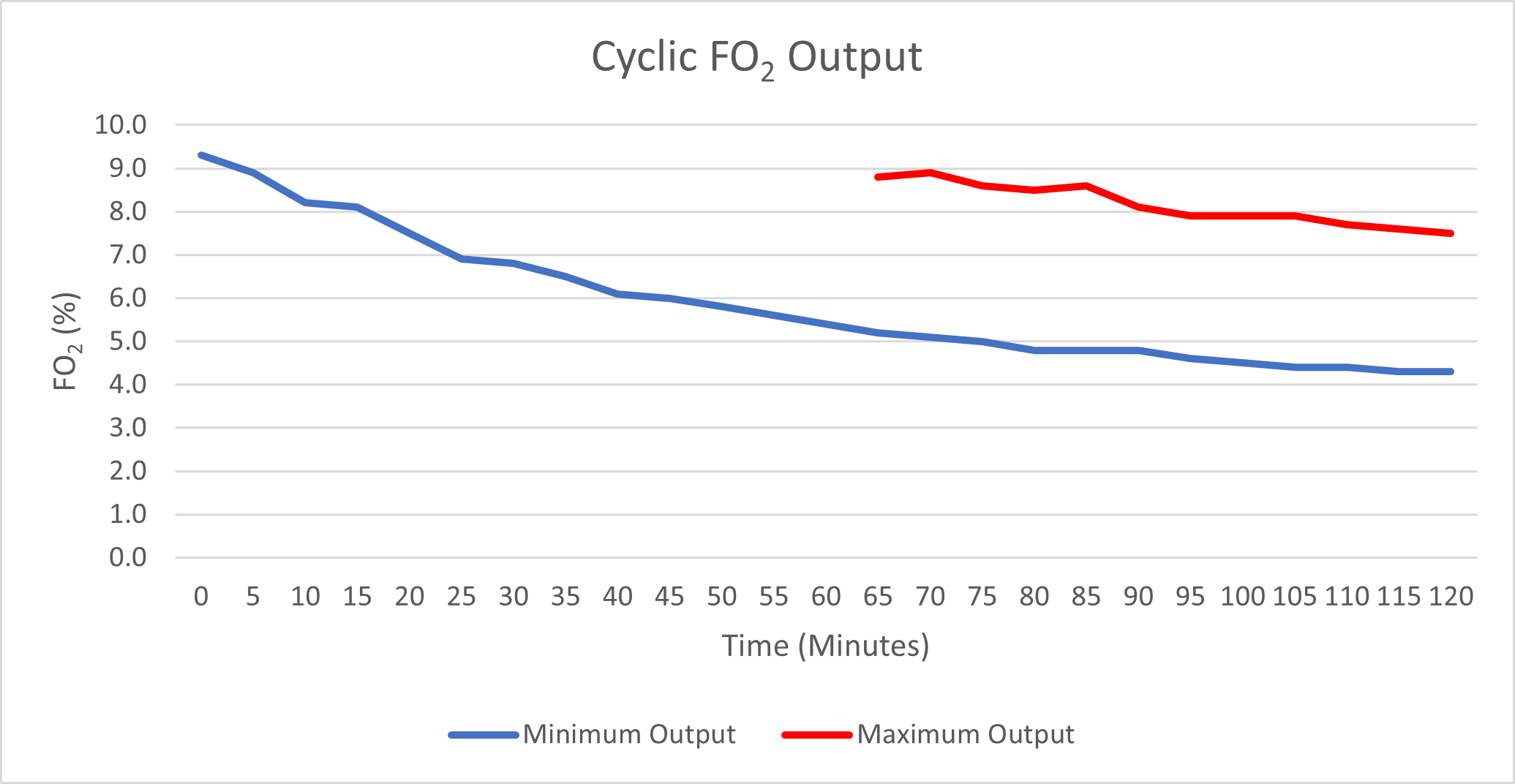
Figure 7: Generator cyclic FO2 output ranges over time while in normobaric extreme hypoxia test configuration 2.
The results of test configuration 2 confirmed with direct evaluation of generator output that operating the JAY-10H within an oxygen-reduced environment results in significant further oxygen depletion, which could be obtained when operating the generator within normobaric normoxic conditions.
Extreme-range NH test configuration 3
The third extreme-range NH experiment represented an extension of experimental configuration 2 but incorporated a 1000-litre reservoir bag attached directly to the generator and placed within the 2080-litre altitude tent enclosure (Figure 8). The reservoir bag outlet was connected to a split tubing system, each fitted with one-way valves. NH output from the reservoir bag was either directed to the subject for on-demand breathing or diverted back into the altitude tent for recirculation and further oxygen depletion. Output FO2 was measured off the test subject line using a low-volume T piece.
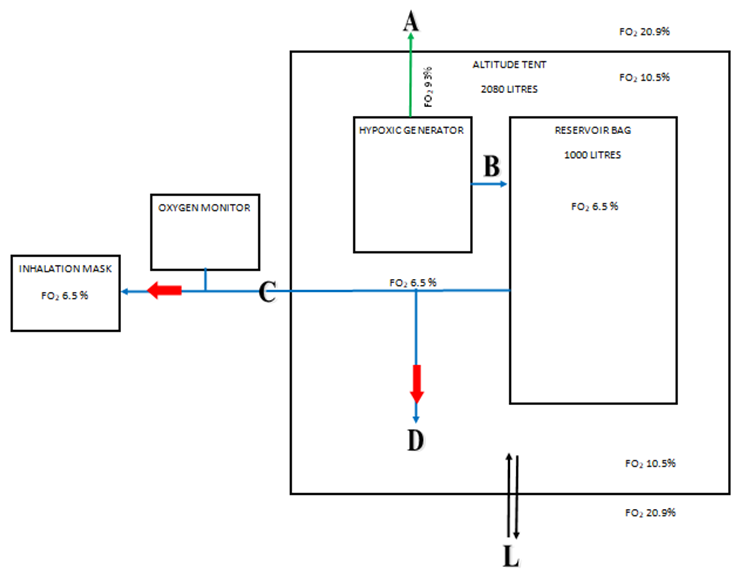
Figure 8: Extreme-range NH test configuration 3. Steady-state conditions for extreme-range normobaric hypoxic chamber in final working configuration. Red arrows: One-way valves with directionality marked. Blue arrows: Movement of depleted oxygen hypoxic air. A (green arrow): Oxygenated waste output from hypoxic generator (FO2 93%) removed from the system into atmosphere. B: Extreme-range normobaric hypoxic air direct from generator with cyclic variation in concentration (FO2 4.3% – 7.5%). C: On-demand reservoir bag output (consistent FO2 6.5%). D: Reservoir diversion recirculating unused hypoxic air into altitude tent for subsequent reuptake by hypoxic generator. L: Two-way leak rate between altitude tent and surrounding atmosphere.
In addition to the advantages of having test subjects outside the altitude tent, attaching a 1000-litre reservoir bag in this configuration creates inspired FO2 consistency by blending the gas output. A further advantage of having a reservoir bag in this configuration relates to the practical aspects of managing the substantially elevated minute ventilation volume anticipated in subjects when exposed to such extremes of hypoxia, which may exceed generator capacity when operating under these conditions.
A consistent NH output FO2 of 6.5% was obtained (PO2 31.4 kPa; simulated altitude 9300 m) using this test configuration 3.
Discussion
These evaluations demonstrate that a commercially available portable NH generator may be employed in alternate configurations to create extreme-range normobaric hypoxic conditions. The application of these techniques may be of potential interest as a surrogate alternative to hypobaric hypoxic testing in the conduct of extreme high-altitude research or for use in training activities where the neurocognitive effects of effect of rapid altitude exposures with minimal preconditioning acclimatisation are being simulated.
These experimental configurations validate the working hypothesis that reducing ambient oxygen partial pressure of air intake into a portable hypoxia generator will result in further depletion of NH air produced by the device. The practical value of configurations developed in these experiments is that they may be easily reproduced using inexpensive equipment readily available on the commercial market.
Further modifications of the configurations described will likely result in further oxygen depletion of the NH output obtained. For example, enclosing the NH generator within a definitively closed loop system and eliminating the leak rate associated with using a standard altitude tent would likely result in further oxygen depletion. Similarly, more powerful higher-flow hypoxic generator systems may be used to offset the impact of leak rate associated with altitude tent enclosures. Such configurations may be of interest to aircrew wanting to achieve simulated altitudes above 9300 m; however, hypobaric hypoxic testing may be a more appropriate and higher fidelity method for such applications.
Conclusion
This manuscript describes the development of an extreme-range normobaric hypoxic environment (FO2 6.5%; PO2 31.4 kPa; simulated altitude 9300 m) using readily available commercial equipment and a standard portable NH generator. The experimental configurations described validate the working hypothesis that reducing atmospheric oxygen partial pressure of air intake into a portable normobaric hypoxia generator will result in further depletion of NH air produced by the device. Within appropriately controlled conditions, obtaining extreme-range normobaric hypoxic environments may be of interest to high-altitude mountaineers and to conduct research activities involving extreme simulated altitudes.
Warning disclosure
Exposure to extreme-range hypoxia using the methods described in this manuscript may result in severe permanent hypoxic brain injury or death. These techniques are recommended for use by trained health professionals and researchers within highly controlled and supervised environments. Under no circumstances is the use of NH for athletic conditioning outside of the indications and methods described by the relevant product manufacturer recommended. The author takes no responsibility for injury sustained by applying any of the techniques described in this manuscript.
Disclaimer
The views expressed in this manuscript are those of the author and do not reflect official Australian Defence Force policy or endorsement.
Conflict of interest statement
The author(s) declare no conflicting interests in relation to this manuscript.
Corresponding Author: Patrick Weinrauch, patrick.weinrauch@defence.gov.au
Authors: P Weinrauch1,2,3
Author Affiliations:
1 Australian Defence Force – 2nd Health Battalion
2 Brisbane Hip Clinic
3 Griffith University Faculty of Health