Abstract
Army has developed a robotic and autonomous system (RAS) strategy (2018); however, health has yet to feature in this domain. Artificial intelligence can be used to augment surgical and resuscitative intervention on the battlefield utilising current heads-up display technology, aligning with three key strategic aims of the RAS document of 1) maximising soldier performance, 2) improving human decision making, and 3) protecting the fighting force.
Real-time monitoring of resuscitative efforts can minimise errors of omission and reduce preventable death rates on the battlefield. Integrating these systems into the receiving medical treatment facility can similarly augment readiness and minimise handover and delays in instituting life-saving interventions upon arrival at higher echelons of care.
Bandwidth and connectivity denial in the contested environment can be overcome by incorporating algorithmic decision support into the local user hardware so the system can function offline. Online access, when available, can link remote clinician resuscitation experts to integral medics providing care on scene.
Evacuation of casualties may also be enhanced using autonomous systems and crewless vehicles, potentially both airborne and ground-borne.
To be future-ready, Army must be able to rapidly adapt to changing battlefield circumstances. Relying on what has worked in the past risks being surpassed by novel approaches that supersede and out-manoeuvre contemporary fighting forces. This applies equally in military healthcare delivery as it does in combat units. Army must anticipate and embrace changes to gain and maintain an advantage in the future operating environment. As is laid out in Army’s Robotic and Autonomous Systems (RAS) strategy (2018):
‘Of particular importance will be considering the impact of systems that can improve the speed and accuracy of the human decision-making cycle.’ (Chief of Army RM Burr)
Of the five fields described in the RAS document as areas in which Army will seek to gain advantage by harnessing technology, two are directly applicable to health service delivery. A third provides the same overarching theme as is fundamental to health—the function of health service delivery in protecting the fighting force. The other two comprise maximising soldier performance and improving decision making.
Real-time monitoring of resuscitation
Of direct applicability to health is the aim of maximising solider performance by integrating fused sensors that intuitively present relevant patient physiological data aiding clinical decision making and thereby reducing the cognitive burden on combat medics (including combat first aiders [CFA] and medic health technicians [HLTH TECH]) delivering tactical combat casualty care (TCCC), particularly during the high-stress context of care under fire. Real-time clinical data on injured soldiers’ vital parameters with prompts to suggest courses of action to treat life-threatening injuries may be delivered to combat medics via heads-up displays (HUD) integrated into ballistic goggles.
These data may also be viewed by remote clinician resuscitation experts (CRE), who can provide live guidance and contribute to decision making in their relatively stress-free environment remote from the battlefield. However, this effect will be limited by network access, and in a contested or congested environment, provision must be made for complete disconnection from online communications. To counter this, protocols and guidelines can be built into these sensor/information sources to aid and improve decision making offline and may be capable of predicting casualty deterioration faster than humans who may be preoccupied or distracted by other tasks as exist in the combat space.
A review of 4 596 battlefield fatalities found that 24.3% were potentially survivable.1 Common causes of potentially survivable death include compressible haemorrhage, tension pneumothorax and airway compromise.2 These areas present specific targets for remote damage control resuscitation.3 Uncontrolled haemorrhage was solely responsible for greater than 80% of US combat deaths during Operation Iraqi Freedom and Operation Enduring Freedom.2 Life-Saving Intervention (LSI) errors of omission related to airway management, pleural decompression and hypotensive resuscitation are common.4 It is crucial that life-threatening conditions are recognised, and the LSIs are provided promptly to improve outcomes.5
Current TCCC guidelines involve sequential control of exsanguinating haemorrhage, managing airway and breathing, assessing haemorrhagic shock and dressing known wounds. Improved outcomes for casualty care due to these evidence-based guidelines have been demonstrated.6 In the initial care-under-fire phase, the care providers are capable of is limited. It is often not feasible to monitor a casualty while returning effective fire, as no means of remotely tracking vital signs of a casualty is currently available. Also, inherent in any resuscitation situation is the potential for error, which is a significant contributor to patient harm. Specifically, in a battlefield setting, the potential for error is increased due to the unique challenges of the austere environment, including care-under-fire scenarios, lack of medical resources, inadequate physiological monitoring capabilities and cognitive overload.
Remote monitoring of the combat casualty will allow the planning of timely casualty extrication. Continuous monitoring of vital signs will facilitate early recognition of deterioration in immediate post-injury period (historically the ‘Golden Hour’) while simultaneously reducing delays for timely field LSI. Improved casualty outcomes are achieved when proper and adequate LSIs are provided immediately, in contrast to delayed reception and resuscitation. Similar, integrated systems can be used to reduce reception and resuscitation errors in receiving medical treatment facilities (MTF).
Real-time monitoring of resuscitation efforts exists in civilian trauma management and is currently being tested and adjusted. These systems may be adapted to satisfy the parameters of improved decision making and maximising soldier performance required in a military context. Technological solutions exist presently and are in the human-trial phase at civilian trauma centres in Australia. Results will be published regarding feasibility and effectiveness, hopefully within the next 12 to 18 months. Adapting and modifying the existing technology to suit a military combat scenario will require hardening vital structures and replacement of civilian with military equipment, such as substituting standard civilian splash-resistant protective eye shields with ballistic googles, for example.
By harnessing these technologies, the Army health system may gain advantage in healthcare delivery and afford increased freedom of manoeuvre by spatially separating experts in trauma management from the practitioner delivering tasks under instruction. In essence, experts in trauma management can be brought to the casualty at the point of injury, essentially at the time of injury, minimising the time for expert medical advice with the ultimate goal of reducing death from preventable causes. The augmented reality provided by a HUD should enhance healthcare delivery while minimising the cognitive burden for the practitioner on scene. Augmentation of the objective force through the insertion of technology of this nature into current force structures leveraging existing CRE within Army aligns with broader RAS objectives and should be pursued in the healthcare sector.
Real-time monitoring of the broader status of a trauma incident
Beyond physiological monitoring of any single individual casualty, real-time battlefield data relevant to the overall trauma reception and management process that technological advancements may provide include: AME platform availability, number, current location and estimated time to destination; location, vital status and number of casualties en route, even details of ongoing enemy action in the area of interest. Clearly, this wealth of information comes at the cost of potential overload for an individual practitioner. Still, it would be of value to commanders (and specifically the J07) and those overseeing complex military operations.
Evacuation strategies
Novel alternatives to evacuation strategies are another area in which RAS may deliver Army advantage in the future operating environment. The RAS Joint Concept Note (2021) does not specifically address medical intervention, focusing initially on warehousing and distribution as key areas in which RAS may benefit. Equally, in healthcare, autonomous systems can improve survivability and reduce human risk when extracting casualties from the battlefield via crewless vehicles, in addition to reducing cognitive load in high-pressure environments of severe trauma management. Rapid evacuation and protection during transportation when injured on the battlefield are inherent in protecting the fighting force.
The current reliance on rotary-wing aeromedical evacuation (RWAME) may be challenged in a future contested airspace. Smaller footprint unmanned aerial vehicles (UAVs) have the additional beneficial qualities of reduced target acquisition area and reduced human casualty potential while maintaining the rapid extraction times achievable using the air route. Limitations arise regarding en-route care of critical patients. They may constrain the nature of appropriate casualty selection for UAV movement. Remote monitoring of casualty clinical condition will be mandatory in this context. Here again, contingency plans for degradation or denial of communications must be considered.
There are UAV currently in service globally that fulfil the basic requirements making them possible candidates for casualty evacuation capability upgrades in terms of optimal size balancing airframe minimisation versus the ability to transport a casualty physically. Although usually intended for other purposes, mainly intelligence, surveillance and reconnaissance (ISR) roles, it would not be unreasonable to propose and consider structural component additions to the undercarriage to provide a hardened shell capable of housing a litter with attached remote monitoring devices to transport appropriate casualties in the future. Required minimal runway dimensions limit the ability to land in the field to retrieve a casualty, but are of no relevance on returning to a role 2 (or 3) MTF if situated near an airfield, as is usually the case. Rotary-wing UAVs may overcome landing zone constraints in the future.
An overview of future battlefield medicine utilising robotic systems and crewless ground vehicles was published in the Australian literature 2014 in the Journal of Military and Veterans Health.7 Essentially, two functions can be provided remotely—extraction and/or treatment. First, and more realistically in the short to medium term, are robotic battlefield casualty extraction vehicles capable of retrieving and transporting injured soldiers over short distances to cover and concealment where TCCC may be effected more safely by human operators. The unique opportunity afforded by robotic extraction vehicles (REV) is this capacity to traverse terrain without cover and potentially in direct sight of otherwise lethal fire or danger where first responders cannot tactically move. This keeps responders out of danger and augments the capabilities of the human element.8
While theoretically very appealing, the practicalities of retrieving a potentially incapacitated or unconscious casualty pose real difficulties yet to be entirely overcome. For example, simpler REV solutions incorporating a sled dragged or towed by a generic robotic platform require the casualty to load themselves onto the sled and are therefore inherently limited. In contrast, more complex dedicated evacuation robots such as the BEAR (Figure 1), capable of loading the casualty robotically, still need to overcome and work around traumatic injuries (such as upper-or lower-limb amputations common in the improvised explosive device era). Additionally, while increased degrees of freedom of the robotic platform facilitate manoeuvrability, these come at the significant cost of increased complexity of control. Finally, although tailor-made for picking up a human casualty, this robotic solution is specific to that one task, thus limiting its wider utility within Army.
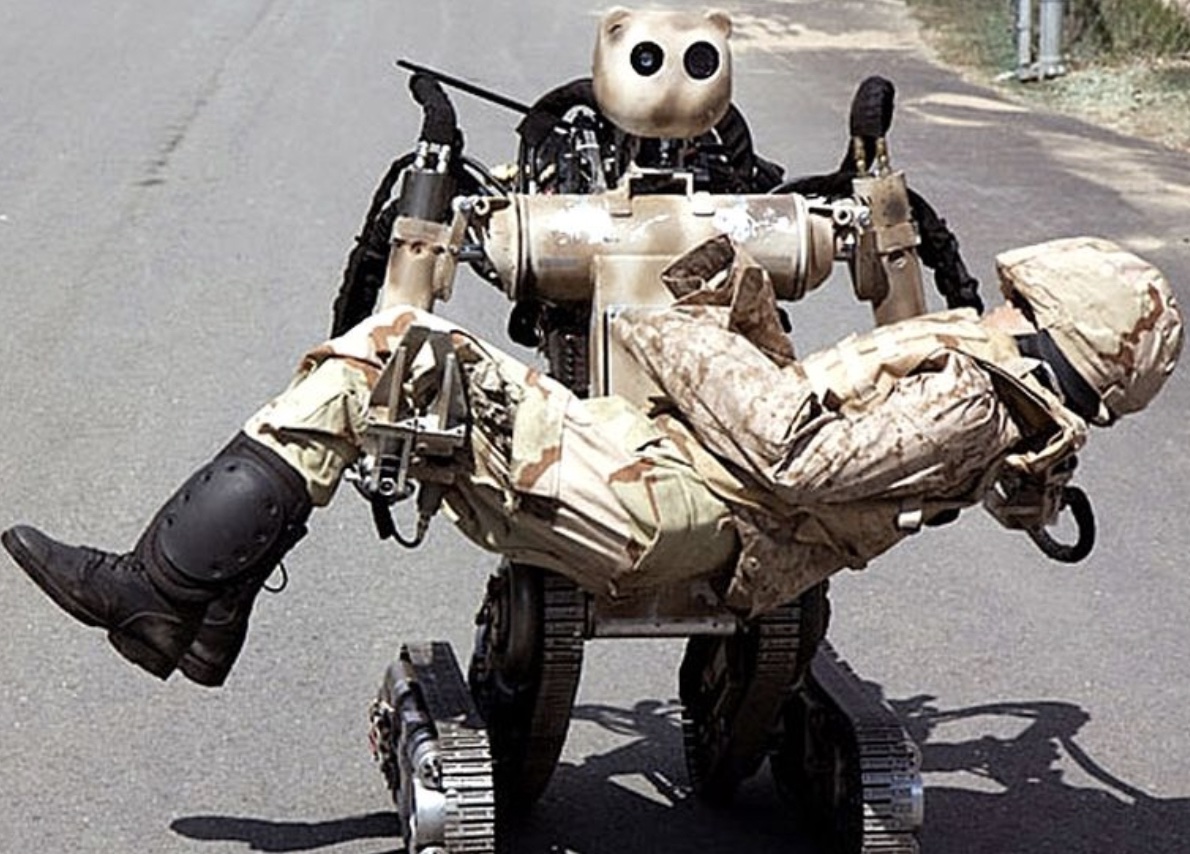
Figure 1 – The BEAR battlefield casualty evacuation robot
Larger and more stable versions of existing land-based crewless vehicles, such as the Ghost Robotics quadruped robot (Figure 2), recently demonstrated at the Robotics Expo and Quantum technology challenge during the Chief of Army Symposium 2021, may potentially be fitted to achieve health effects of patient ground extraction capabilities in future iterations. Similar to the fit-out required to equip an UAV to transport a casualty, some form of hardened litter shell that could open along its length to receive and then close around a casualty for protection could be developed. This modular structure could be fitted to a generic robot dog in general service, temporarily repurposing it for casualty evacuation opportunistically. This structure is envisaged to be attached to the undercarriage of the torso of the robotic dog coupled with an internal four-pronged claw to slide under, lift and secure the casualty, simplifying patient loading and protection during transportation. The major attraction of options such as this is the multiple configurations of the robotic platform available, broadening the appeal of the associated capital expenditure that can deliver multiple effects on the battlefield. However, significant work would be required to develop a functional casualty loading device such as that postulated here. As it stands, the optimal robotic platform for casualty evacuation remains to be realised but remains a significant opportunity for robotics to influence healthcare delivery in the future operating environment.
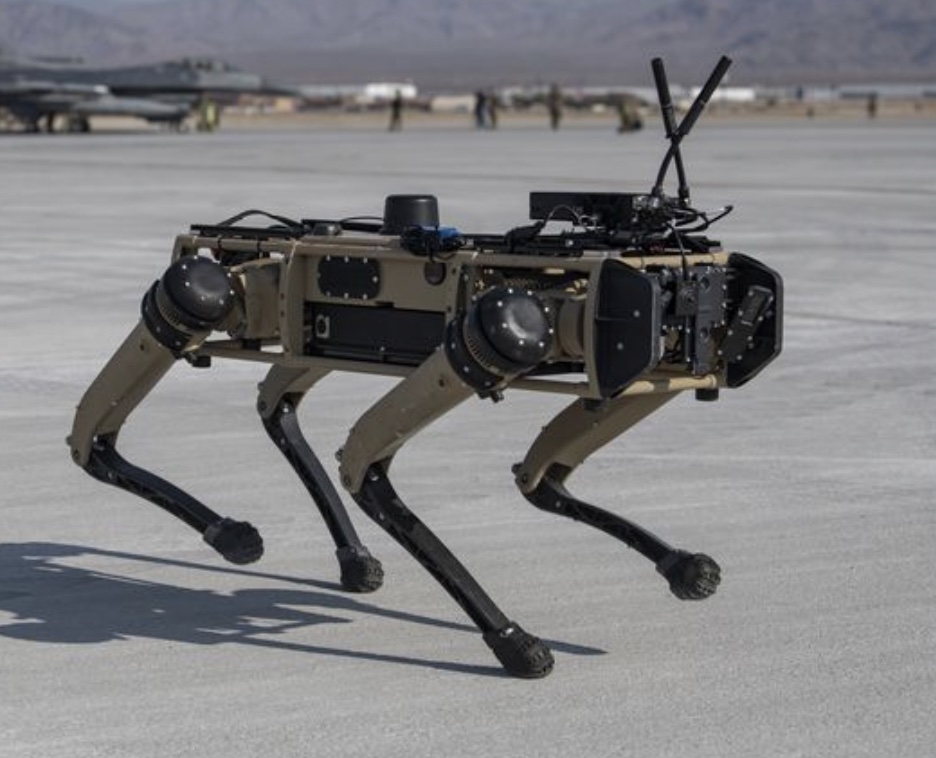
Figure 2 – Ghost quadruped robotic dog
Robotic platforms capable of delivering TCCC interventions are also being evaluated. However, these may be made redundant by REV, which can promptly transport a casualty back to a human operator.
Ideally, advanced REV should also be able to provide the receiving MTF with an enhanced report of incoming casualties by feeding live monitoring from evacuation platforms relaying integrated patient physiological sensor data. Based on this information, anticipation and preparation for necessary procedures can be planned with more clarity. In addition, they should improve casualty movement by directing or redirecting more or less acute cases to appropriate facilities where capacity exists. For example, deterioration indicating urgent neurosurgical intervention may redirect a casualty to a role 3 MTF from an initial role 2E destination.
Robotic/remote surgery
Further into the future, robotic surgery (which is of increasing interest in the civilian elective surgery setting) may yet be able to deliver remote surgery options in the forward environment. The civilian industry leader Intuitive with the Da Vinci system™ was initially developed with a military setting in mind with grant support from NASA and the US Defense Advanced Research Projects Agency but still requires a surgically skilled operator at the patient bedside. Additionally, the current infrastructure required to effect robotic surgery is incompatible with military deployment. The key benefit of a robotic platform of this nature is that, with sufficient bandwidth, a surgical procedure can be performed by any surgeon anywhere in the world utilising the robotic system once docked to the patient. Therefore, the bedside operator on deployment theoretically only requires the skillset to obtain access to the patient and to dock the robot, while more advanced surgical practitioners may be located anywhere in the world, well away from the battlefield and any threat. However, the biggest limitation to the further progression of remote robotic surgery in the military context is the time-critical nature of damage control trauma surgery. This requires maximal and rapid access to bodily cavities where torrential uncontrolled haemorrhage may occur, obscuring vision through anything other than a major incision, leaving no role for the minimally invasive approach. Therefore, robotic surgery is not appropriate for damage control trauma surgery.
Summary
Efficiency in trauma healthcare delivery may be obtained by embedding RAS in the pre-hospital setting in the contexts of immediate trauma resuscitation at the point of injury, and through enhanced patient evacuation. Trauma resuscitation may be optimised with a greater range of medical interventions delivered by practitioners augmented with HUD under instruction by CRE, backed up by algorithmic analysis of real-time alterations in physiology cueing medical intervention when necessary. Autonomous medical evacuation vehicles with a live feed of vital parameters to alert and prepare the receiving MTF should also improve patient outcomes, further minimising death and disability from trauma and is the future of healthcare in the autonomous systems age.
To paraphrase the Chief of Army, the increased use of RAS capabilities will fundamentally change the way Army delivers effects on the battlefield (including in the healthcare domain) by increasing situational awareness, reducing soldier cognitive workload and increasing the reach and range of health service capabilities. Therefore, it should be prioritised to maintain a competitive advantage over our adversaries.
Disclaimer: The views presented here are those of the authors and do not represent the views of the Directorate of Army Health or the Australian Army.