K Tillotson, LM Wentz, M Roy, CS Berry-Caban
Abstract
Background: Traumatic brain injury (TBI) has been shown to cause pituitary dysfunction, manifesting in low testosterone concentrations, and previous research suggests a link with vitamin D deficiency.
Purpose: To compare testosterone and vitamin D concentrations in service members with and without a TBI,
and to identify the frequency of testosterone prescriptions.
Materials and methods: This retrospective de-identified medical review analysed assessments (testosterone, vitamin D) ordered for 4285 active duty and veteran military personnel at Womack Army Medical Center, Fort Bragg, NC from 2016–2018.
Results: Overall, 343 (8%) of service members had a medically diagnosed TBI. In all men, 19% were deficient in testosterone (<270 ng/dl), and 10% had a testosterone prescription. Active duty men with TBI history had lower testosterone than active duty men with no documented head injury (431 ± 162 vs 452 ± 170 ng/dl, P = 0.04). However, there was no significant difference in veteran men. More than one-third (38%) of all service members were insufficient in vitamin D (<30 ng/ml). Overall there was a weak positive correlation between testosterone and vitamin D concentrations in men but not in women.
Conclusions: Our research does not support evidence for high rates of hypogonadism, testosterone prescription, or vitamin D deficiency after TBI compared to military personnel without prior injury. However, we found a high prevalence of vitamin D insufficiency in active duty and retired service members independent of TBI, further supporting that vitamin D status should be assessed regularly in service members.
Key words: traumatic brain injury; vitamin D; testosterone; testosterone replacement therapy; military personnel Conflict of Interest: No funding was secured for this study.
Acknowledgments: We appreciate the assistance of the Womack Army Medical Center Information Management Division for data acquisition and Dr Melissa Gutschall for her edits and serving as a thesis committee member.
Introduction
Traumatic brain injury (TBI) causes long-term consequences, including physical disabilities and behavioural, cognitive and psychological defects that may prevent military personnel from performing to their greatest potential and negatively impact long-term health. Nearly half of TBI cases result in neuroendocrine dysfunction, characterised by hormone imbalances directly related to the hypothalamus and pituitary gland, and their axes. Disruptions to the endocrine axes are caused by an inadequate supply of hypothalamic-releasing hormones or the pituitary gland’s inability to produce hormones, both of which result in pituitary dysfunction. Hypopituitarism with gonadotropin deficiency is one of the most prevalent types of neuroendocrine dysfunction following TBI and manifests as low testosterone concentrations. In the immediate post-TBI period, low testosterone concentrations have been observed in up to 80% of individuals but resolve in the majority during the chronic phase 3 months after injury. While neuroendocrine disorders have been well-established post-TBI, vitamin D’s role is poorly understood, as evidence suggests vitamin D deficiency may be associated with TBI sequelae. Vitamin D’s neuroprotective role following TBI has been explored in several studies, most of which have used animal models. It is unclear if TBI causes low vitamin D, or if low vitamin D prior to TBI exacerbates injury. Numerous studies have shown a decrease in testosterone or vitamin D concentrations following TBI in civilians. Clinical hypopituitarism has been observed in all TBI severities. Hypogonadism and growth hormone deficiency are typically the most common pituitary abnormalities, although testosterone concentrations vary based on injury severity. Four studies have investigated testosterone status in military personnel following mild, moderate, and severe blast-related TBI but did not report differences between severities. Overall, these studies found low testosterone following injury, and one study found a significant decrease in testosterone three years after injury compared to controls. Civilian studies support high prevalence of vitamin D deficiency post-TBI, with one researcher identifying the lowest vitamin D concentrations in the most severe cases of TBI. A few studies have established a positive correlation between testosterone and vitamin D concentrations in men, possibly due to the expression of the vitamin D receptor and metabolising enzymes in the Leydig cells of the testes. Furthermore, Pilz et al. found vitamin D supplementation in deficient males significantly increased testosterone compared to a placebo group. These data suggest that vitamin D has a supporting role in regulating testosterone production.
No research to date has examined both testosterone and vitamin D status together after TBI in military personnel or civilians, despite the evidence that these hormone deficiencies may exacerbate postconcussive symptoms. Furthermore, research examining the use of testosterone replacement therapy following TBI is limited. Therefore, the purpose of this study was to compare testosterone and vitamin D concentrations between service members with and without a history of TBI and to identify rates of testosterone replacement therapy at a large military treatment facility. Characterising the incidence of TBI-related hypogonadism and vitamin D deficiency in military personnel helps to identify targets for therapeutic treatments to improve longterm recovery.
Methods
Study design and procedures
This retrospective de-identified medical review investigated testosterone and vitamin D status in active duty and retired service members, with and without a history of TBI, and the frequency of testosterone replacement therapy prescriptions.
Study population
The study population included 4285 veteran and active duty service members from the United States (US) military who had serum testosterone and vitamin D ordered and assessed at Womack Army Medical Center (WAMC), Fort Bragg, NC between October 2016 and December 2018. Appalachian State University Institutional Review Board approved this protocol, and a letter of agreement was established with WAMC. Inclusion criteria were current or previous military service, male or female, aged >18 years, with serum testosterone assessment. Vitamin D was included in analysis if assessed at the same time as testosterone. Additional data collected from medical records included diagnosis of TBI, prescription for testosterone replacement therapy, date of hormonal assessments, and participant demographics: age, gender, and active duty/veteran military status.
Data analysis
All analyses were conducted with the statistical software package, Stata 15 (StataCorp 2017). Independent t-tests were used to identify differences between continuous variables. Chi-square tests were used to identify differences between dichotomous variables. A one-way ANOVA with Bonferroni posthoc analysis was used to test for differences in vitamin D across seasons. Statistical significance was P < 0.05.
Results
Traumatic brain injury in service members
From 2016–2018, 4285 unique cases with testosterone assessments were identified, of which 3204 (75%) of participants were men, and 2675 (62%) were active duty. Overall, 343 (8%) of service members and veterans were diagnosed with a TBI between October 2016 and September 2018. One hundred and ninety-eight service members had a mild or moderate TBI diagnosis, while 143 service members had a TBI of unknown severity. Active duty personnel had higher rates of TBI compared to veterans (12 vs 2%, χ2 (1, n = 4285) = 124, P < 0.01). Men were significantly more likely to be diagnosed with a TBI than women (10 vs 1%, χ2 (1, n = 4285) = 93, P < 0.01).
Testosterone concentrations in service members
Overall mean total testosterone concentrations were 427 ± 179 ng/dl (range 5–800 ng/dl) for men and 31 ± 35 ng/dl (range 5-636 ng/dl) for women (Table 1). Of 3204 men who were assessed, 19% were deficient in testosterone (<270 ng/dl) according to the Army Medical Department Clinical Guidelines, and 25% were deficient according to the American Association of Clinical Endocrinologists Medical Guidelines22 (<300 ng/dl); 10% of men had a testosterone prescription. Active duty men without testosterone prescriptions had significantly higher testosterone concentrations than active duty men with prescriptions (456 ± 163 vs 365 ± 225 ng/dl, t = 5, P < 0.001). As expected, active duty men had higher testosterone concentrations, lower rates of testosterone deficiency (by both clinical cut-offs), lower rates of testosterone prescriptions, and were younger compared to veteran men (Table 1). Women were younger with lower testosterone concentrations and lower rates of testosterone prescription than men. Of the 1081 women with testosterone assessments, n = 5 (3 active duty, 2 veteran) had high testosterone concentrations (279–636 ng/dl) but did not have testosterone prescriptions. Four women (1 active duty, 3 veteran) were prescribed testosterone, with similar formulations as those prescribed to male military personnel in this sample. Testosterone prescriptions consisted of gels, pellets, patches and injections of low and high doses (range 2.5–200 mg of testosterone). However, the exact dose prescribed per day is unknown. In men with a history of TBI, 15% (<270 ng/dl) and 22% (<300 ng/dl) had testosterone deficiency. By comparison, men without TBI had higher rates of testosterone deficiency (19% <270 ng/ dl; 25% <300 ng/dl). This difference was statistically significant for testosterone <270 ng/dl only (χ2 (1, n = 3204) = 4, P = 0.039). There were no differences in testosterone prescriptions between men with and without TBI (7 vs 10%, χ² (1, n = 3204) = 3, P = 0.068). Although total testosterone concentrations among all men (active duty and veteran) did not differ based on TBI history, active duty men with a history of TBI had lower testosterone compared to active duty men with no documented head injury (Figure 1; 431 ± 162 vs 452 ± 170 ng/dl, t = 2, P = 0.035). This difference was not significant in veteran men (Figure 1; 418 ± 138 vs 353 ± 190 ng/dl, t = –2, P = 0.07). When men with testosterone prescriptions were excluded from analysis, active duty men with a history of TBI still had lower concentrations of testosterone than active duty men without TBI but not at a significant level (440 ± 158 vs 458 ± 163 ng/dl, t = 2, P = 0.07). There were no significant differences in testosterone concentrations in women with and without a history of TBI (28 ± 20 vs 31 ± 35 ng/dl, t = 0.50, P = 0.66).
Table 1. Service member’s testosterone, vitamin D, and history of TBI by sex and military status
Active Duty Men (n = 2427) |
Veteran Men (n = 777) |
Active Duty Women (n = 248) |
Veteran Women (n = 833) |
|
---|---|---|---|---|
Age (yrs) | 37 ± 8AC | 54 ± 12B | 32 ± 8B | 33 ± 10 |
TBI | n = 301 (12%)ABC | n = 30 (4%) | n = 9 (4%) | n = 3 (0.4%) |
Testosterone (ng/dl) | 450 ± 169ABC | 356 ± 189AB | 33 ± 42 | 30 ± 32 |
Testosterone Deficiency (<270 ng/dl) | n = 340 (14%)C | n = 259 (33%) | ||
Testosterone Prescriptions | n = 169 (7%)ABC | n = 155 (20%)AB | n = 1 (0.4%) | n = 4 (0.5%) |
Vitamin D (ng/ml)† | 35 ± 12A | 35 ± 11B | 33 ± 11 | 32 ± 12 |
Vitamin D Insufficiency† (<30 ng/ml | n = 365 (35%) | n = 127 (38%)B | n = 39 (45%) | n = 127 (47%) |
Data are presented as mean ± standard deviation for continuous variables †Not every subject had a vitamin D value (n = 1742). AP < 0.05 vs active duty women. BP < 0.05 vs veteran women. CP < 0.05 vs veteran men. Active duty men had lower rates of testosterone deficiency using AACE Medical Guidelines (<300 ng/dl) compared to veteran men (19 vs 42%, χ2 (1, n = 3204) = 166, P < 0.001).
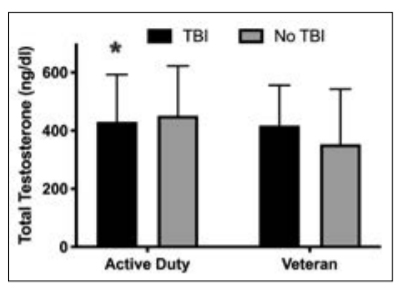
Figure 1. Testosterone concentrations in active duty and veteran men with and without TBI. Data are presented as means ± standard deviation. *P < 0.05 significantly lower than active duty males without TBI.
Vitamin D concentrations in service members
Overall, mean vitamin D concentrations were 35 ± 12 ng/ml (range 8–60 ng/ml) for men and 32 ± 12 ng/ml (range 9–60 ng/ml) for women. Thirty-eight per cent of all service members assessed were insufficient in vitamin D (< 30 ng/ml; 36% of men, 46% of women).
One hundred and fifty-four service members (9%) were deficient in vitamin D (< 20 ng/ml). Active duty service members had higher concentrations of vitamin D compared to veterans (35 ± 12 vs 33 ± 12 ng/ml, t = –3, P = < 0.01). Men had higher vitamin D concentrations compared to women (35 ± 12 vs 32 ± 12 ng/ml, t = –4, P = < 0.001). Active duty men had higher vitamin D concentrations than active duty women, and veteran men had higher concentrations of vitamin D than veteran women (Table 1). There were no significant differences in vitamin D concentrations between active duty and veteran men or between active duty and veteran women. Veteran women were significantly more likely to have vitamin D insufficiency than veteran men, although there was no difference between active duty men and active duty women. The seasonal vitamin D assay was evenly distributed—assessments were 24% in the spring, 23% summer, 29% autumn and 24%
winter. Vitamin D concentrations were significantly different across seasons, hitting a nadir in winter and peak in summer (F(3, 1738) = 26, P < 0.001; Figure 2). Vitamin D concentrations in winter (32 ± 11 ng/ml) were significantly lower than spring (35 ± 12 ng/ml), summer (38 ± 12 ng/ml) and autumn (34 ± 11 ng/ml, P < 0.001). Summer vitamin D concentrations were higher than in the spring and autumn, but there were no significant differences between autumn and spring. Contrary to our hypothesis, service members with a history of TBI had significantly higher concentrations of vitamin D compared to service members without a TBI (36 ± 12 vs 34 ± 12 ng/ml, t = –2, P = 0.034), but the difference was minimal (2 ng/ml) and of little clinical significance. There was no significant difference in service members with and without TBI for vitamin D insufficiency (34 vs 38%, χ2 (1, n = 1742) = 1, P = 0.26). Vitamin D concentrations were not different between active duty or veteran service members with or without TBI history (Figure 3).
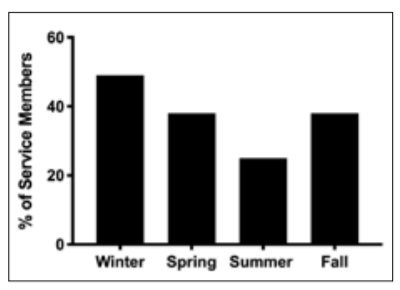
Figure 2. Percentage of Service Members Insufficient in Vitamin D (<30 ng/ml) Across Each Season (in the US): Winter (December, January, February), Spring (March, April May), Summer (June, July, August), Autumn (September, October, November).
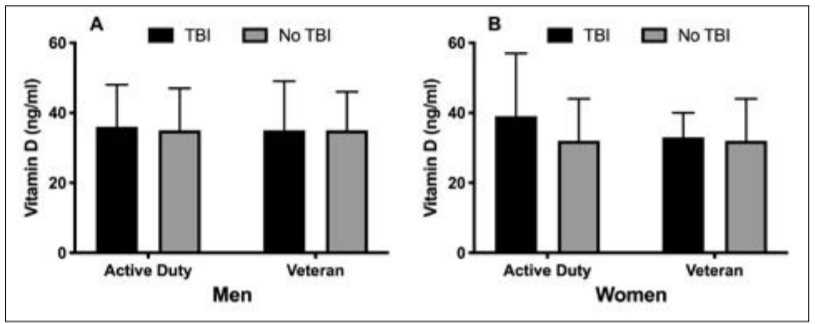
Figure 3. Vitamin D concentrations in active duty and veteran men (A) and women (B) with and without TBI. Data are presented as means ± standard deviation. There were no significant differences between groups.
Correlations between testosterone and vitamin D concentrations in service members
Overall, there was a weak positive correlation between testosterone and vitamin D concentrations for men (r = 0.099, P < 0.001) but not for women (r = 0.004, P = 0.94). This significant correlation was maintained when compared among active duty men (r = 0.087, P = 0.005) and veteran men (r = 0.12, P = 0.028). When men were analysed separately by testosterone prescription, there was still a positive correlation between vitamin D and testosterone concentrations for men without testosterone prescriptions (r = 0.095, P = 0.003). No significant correlations were found in active duty or veteran women. There was a weak positive but significant correlation between testosterone and vitamin D concentrations (r = 0.099, P < 0.001) for men without a TBI compared to men with a TBI (r = 0.11, P = 0.12) Likewise, active duty men without a TBI demonstrated a weak but significant positive correlation between testosterone and vitamin D concentrations (r = 0.081, P = 0.018), while active duty men with a history of TBI had no significant correlation (r = 0.13, P = 0.076). No significant correlations were found in active duty or veteran women with a TBI.
Discussion
Traumatic brain injury has been shown to cause low testosterone concentrations due to pituitary dysfunction and may affect vitamin D status, but no published research to date has investigated both of these hormones in military personnel with TBI. In this retrospective analysis, we did not find higher rates of pituitary dysfunction (low testosterone) or vitamin D deficiency in military personnel with a TBI history compared to military personnel without prior injury. In our dataset, active duty men with a TBI had statistically lower testosterone than active duty men without prior injury, but the difference was small and of little clinical significance. No clinically meaningful relationship was observed between TBI and vitamin D deficiency. However, our overall dataset showed a high prevalence of vitamin D insufficiency in active duty and retired service members independent of TBI. Correlations between testosterone and vitamin D concentrations were positive but weak in men. Perhaps these findings are driven by a low medically reported TBI diagnosis. Eight per cent of military personnel in our sample had a TBI diagnosis, which is lower than previous research showing 11–23% of military personnel had been diagnosed with a TBI. Our overall findings in military personnel do not support high prevalence of hypogonadism in men with a history of TBI. Previous research has found pituitary dysfunction in 31–48% of male service members after TBI, although not exclusive to hypogonadism. In our sample, 15% of men had low testosterone, which is comparable to other reported findings of hypogonadism in service members ranging from 0–19%.2,16-18 Contrary to military studies, civilian studies investigating pituitary dysfunction after TBI have shown higher hypogonadism rates among participants, ranging from 1.4–80%. These findings suggest that blast-induced TBI may affect pituitary function differently from non-blast-induced TBI, resulting in lower hypogonadism rates. Time post-injury and clinical cut-offs for testosterone deficiency also varied between both military and civilian studies. Nearly all military studies used the 5th or 10th percentile of the sampled group to determine hormone deficiency (199–330 ng/dl). We analysed our data using the Army Medical Department cut-off of 270 ng/dl and the American Association of Clinical Endocrinologists22 and Endocrine Society Clinical Practice Guidelines23 cut-off of <300 ng/dl without significant differences in results. Our results could have been influenced by the time between injury and the testosterone assessment, as we did not have data for this analysis. Gonadotropin function has been shown to spontaneously resolve at 3 to 12 months after injury in a majority of patients due to temporary pituitary dysfunction from hypothalamic-pituitary oedema, increased intracranial pressure and the physiologic response to either critical illness or drugs used in the acute phase of TBI. Our military personnel with TBI may have had testosterone deficiency but recovered prior to testosterone assessment and treatment, which may explain our low rates of hypogonadism. In addition, a negative correlation between testosterone concentrations and injury severity may exist in the immediate post-TBI period. Perhaps more significant correlations would be evident in severe cases. 24 To our knowledge, this is the first study investigating testosterone prescription after TBI. We found no association between testosterone prescriptions and TBI diagnosis, which would coincide with the low rates of hypogonadism, thus warranting fewer prescriptions. Military treatment facilities have also become more conservative with prescribing testosterone replacement therapy.
Inadequate sleep, energy deficits and physical stress during deployment and Special Operations Forces training have been shown to suppress testosterone levels in military personnel. This finding may explain why active duty men with TBI had lower testosterone concentrations than active duty men without injury, while veterans with TBI did not have this result. Testosterone production may be
reduced post-TBI due to inflammatory cascade cytokines suppressing Leydig cell function in the testes, leading to hypothalamic-pituitary-gonadal axis dysfunction. Veterans with a TBI had higher testosterone than veterans without a TBI, but several veteran men without a TBI in our analysis had very low testosterone (<10 ng/dl). This result is drastically lower than the age-related decline NHANES data has shown with participant’s testosterone concentration at age 80 being 30% less than at age 20.
Interestingly, none of these testosterone deficient veterans were prescribed testosterone replacement therapy; assuming they did not show symptoms of androgen deficiency. This finding would be normal according to the Endocrine Society recommendations.
Another explanation for this finding could be influenced by the unknown length of time after injury since anterior pituitary trauma may result in normal or high serum concentrations of testosterone from the acute release of stored hormones after injury.
Our findings on vitamin D concentrations support previous research in civilians and military personnel, showing that low vitamin D is a common ailment independent of TBI. To our knowledge, this is the first study examining both testosterone and vitamin D concentrations in military personnel with and without TBI. Previous studies of civilians after TBI found similar vitamin D deficiency rates compared to our results (24–47% vs 34%). However, the cut- off rates for these studies were lower than ours with < 10, 14 and 16 ng/ml being deficient. Two studies with an equivalent cut-off found much higher rates of insufficiency at 63–95%. Rates of deficiency/insufficiency are influenced by differences in latitude, clothing, race and diet of the different countries in which these studies were conducted. The 35.1°N latitude of Fort Bragg allows for an extended period of endogenous vitamin D synthesis that would explain our lower rate of vitamin D insufficiency versus other studies. We did not have adequate racial data to include in the analysis.
Studies have shown mixed results between vitamin D concentrations and TBI severity. Jamall et al. 12 found no difference in vitamin D among TBI severities, while Toman et al. 13 found patients with severe TBI were the most deficient in vitamin D. Separate analysis of the most severe TBI cases may have shown a higher prevalence of vitamin D deficiency. However, we did not have severity data for all cases. In this study, the seasonal distribution of vitamin D assessments was fairly evenly distributed throughout the year. In the US, vitamin D seasonality peaks in August and nadirs in February; and during winter months ultraviolet radiation for most US latitudes north of Atlanta, GA (33.7°N) is inadequate for sufficient endogenous synthesis of vitamin D.
Our weak but positive correlation between vitamin D and testosterone in servicemen is consistent with previous findings by Wentz et al.
19, who found a stronger correlation in vitamin D deficient men. Contrary to these findings, one study found an increase in testosterone concentrations in active duty males during basic military training despite a significant decrease in vitamin D. The intense training and anabolic adaptations that occur during basic military training plus the younger age of soldiers who participate in bootcamp may explain the different correlations between vitamin D and testosterone. Vitamin D may be positively correlated with testosterone concentrations in men due to the expression of the vitamin D receptor and metabolising enzymes in the Leydig cells of the testes and has been shown to raise testosterone levels in vitamin D deficient men. No correlation was found between vitamin D and testosterone in men with TBI, possibly due to a smaller sample size given that no difference was discovered in vitamin D between men with and without injury.
We did not find evidence that TBI causes low vitamin D, but other research suggests that low vitamin D may exacerbate injury. Vitamin D may promote resilience after TBI by regulating calcium ions, oxidative stress, inflammation and apoptosis during the secondary cascade of injury. Cui et al. found that calcitriol treatment can improve neurobehavioral defects and cerebral oedema in rats after TBI. In human trials, one study found patients with vitamin D deficiency on admission to the neurological critical care unit had worse three-month Glasgow Outcome Scores than vitamin D sufficient patients. Another study found vitamin D supplementation increased long – term performance and cognitive outcomes in vitamin D deficient patients with mild to moderate TBI.
Our overall dataset shows a high prevalence of vitamin D insufficiency. Since military personnel are at high risk for TBI and tactical gear limits adequate sunlight exposure, it is recommended to assess vitamin D status biannually. It would be beneficial to supplement those with insufficiency and deficiency accordingly to Endocrine Society Guidelines to maintain optimal vitamin D levels as a preventive measure to improve resiliency post-TBI. Since gonadotroph function may spontaneously recover in 3 to 12 months after injury, endocrine evaluation should be completed at 3 to 6 months and re-assessed at 12 months post-TBI. Studies have shown testosterone replacement therapy to increase lean body mass and strength while reversing cachexia in diseased populations and readily reverse symptoms of neuroendocrine dysfunction once identified.
This study was strengthened by a large set of medical records reviewed for one geographical location, which limited the effect of latitude. This study’s limitations include the medical record review’s observational nature in which causal relationships cannot be established, only correlations. Furthermore, data for confounding variables such as race, sex hormone binding globulin, training status, dietary supplements, testosterone prescriptions prescribed by providers outside of the DoD medical system, length of time after injury, and body mass composition were not available for analysis. Lastly, this sample consists of military personnel who had testosterone and vitamin D ordered by a physician and is not representative of all service members.
Our research does not support evidence for high rates of hypogonadism, testosterone prescription or vitamin D deficiency after TBI compared to military personnel without prior injury. However, we show a high prevalence of vitamin D insufficiency in active duty and retired service members independent of TBI, further supporting that vitamin D status should be assessed regularly in service members. A prospective analysis pre-injury may provide better insight into the role of testosterone and vitamin D in TBI.
Disclaimer
The views expressed herein are those of the authors and do not reflect the official policy of the Department of the Army, Defense Health Agency, Department of Defense or the US Government.
Corresponding Author: Laurel Wentz wentzlm@appstate.edu
Authors: K Tillotson, L Wentz, M Roy, C BerryCaban
Author Affiliations:
1 Appalachian State University, Department of Nutrition and Healthcare Management
2 Womack Army Medical Centre, Clinical Investigation