Introduction
The rescue of severely wounded soldiers, while under fire, is itself a major cause of military death and traumatic injury.1 Some sources estimate that up to 86% of battlefield deaths occur after the first 30 minutes post-injury.1,2 Hence life saving training techniques3 and treatments, and more recently, the application of robotic surgical systems (RSS; Fig.1), technologies and unmanned vehicles (UVs), have been developed to provide battlefield casualty extraction, critical life-saving interventions, and physiological monitoring, in order to reduce this incidence. Although not invincible themselves, when it comes to enemy small arms fire, UVs and RGPs can sustain a lot more direct fire than can the average human soldier, hence their utility in combat first responder scenario’s.
Just as unmanned aerial vehicles (UAVs)4 have continued to provide grounds troops with timely intelligence, surveillance and reconnaissance capabilities, and when armed, with the ability to bomb enemy targets using precision-guided bombs, today, unmanned ground vehicles (UGVs) and robotic ground platforms (RGPs), are increasingly being developed. Not only to search for improvised explosive devices, but also as important battlefield life-saving technologies. With today’s battlespace domination by various ‘life-taking’ weaponised robots, which can achieve ‘lethality via remote- control’, it has been encouraging to see the recent proliferation and availability of new ‘life-preserving’ technologies and unmanned platforms.
Over recent years, these technologies have grown smaller, lighter, faster, more agile and sophisticated. While UAVs to date have featured most prominently in the air, state-of-the-art RSS, UGVs and RGPs are proliferating, and are being increasingly used. Such technologies include surgical robots, ‘porter’ or load- carrying UVs and battlefield casualty extraction devices (both air and ground). The latter include the development of UAVs specifically designed for casualty air-lift evacuation (though these are not covered here). This article describes the advances, variety and utility of some RSS, UGVs and RGPs that have potential application for use in battlefield medicine, and outlines some current systems and prototype models in the testing phases.
On-site Robotic Surgical Systems
The idea of RSS, or technologies that use robotic systems to aid in surgical procedures on-site, have been around for over three decades. In 1992, Dr. Senthil Nathan of Guy’s and St. Thomas hospital in London successfully carried out the first robotic surgical procedure (prostatectomy) in the world, using ‘Probot’, developed at Imperial College London. Since then, RSS development was advanced further by two companies working together, SRI International and Intuitive Surgical, who had introduced the ‘da Vinci’ surgical system as well as ‘Computer Motion’ with the ‘AESOP’ and ‘ZEUS’ RSS.5 The ZEUS was later used to perform a Fallopian tube reconnection (July 1998), a beating heart coronary artery bypass graft (Oct. 1999), a closed-chest beating heart cardiac hybrid revascularisation (Nov. 1999) and the ‘Lindbergh (cholecystectomy)operation which was performed remotely (Sept. 2001).6
With grant support from both NASA and DARPA (US Defence Advanced Research Projects Agency), and thanks to the years of pioneering work of Dr. Robert M. Satava, the original telesurgery robotic system was developed, based on the da Vinci design.7 It turned out to be more useful for on-site minimally invasive surgery (MIS), than remotely-performed surgery on the battlefield and other environments. Today, both on-site and remotely-operated RSS have been developed in various shapes and sizes to overcome the limitations of MIS and to enhance the capabilities of surgeons performing open surgery.8 This has also provided the ability to perform ‘remote surgery’ or ‘unmanned surgery’, though at this point in time, this still requires a human operator assisting at the robot end. Of course, this may provide useful applications in settings where highly skilled medical resources are not available such as the battlefield, isolated enclaves, and even space travel.
Robotic surgery is a method to perform surgery using small surgical instruments attached to robotic arms. RSS can be divided into three separate categories contingent upon the level of surgeon interaction during the procedure; these include Supervisory-Controlled, Telesurgical and Shared- Control methods.8 The Supervisory controlled method exclusively employs a robot to perform the entire procedure, which it does in accordance with the computer program loaded into it by the surgeon pre-operatively. The disadvantage of this system is that it must be individually programmed, making it expensive as several images and data for patients are often required. Also known as ‘remote surgery’ the telesurgical method is where a (human) surgeon directly manipulates the robotic arms during a procedure, as opposed to the robotic arms working themselves from pre-loaded software. Using telesurgery, the surgeon can operate from a remote location using sensor data, and real-time image feedback from the robot.8 As an example of this, in 2001, using Computer Motion, the first transatlantic remote surgical intervention was
performed by a doctor in New York, who had removed the gallbladder from a patient located in Strasbourg, France.9 This operation demonstrated that surgery over long distances was indeed possible. The sharedcontrol RSS allows for jointly performed tasks to be undertaken. For example, the robot steadies manipulation of the fine instruments while the surgeon carries out the procedure.8camera built into da Vinci. Proponents assert that the advantages of RSS, are that they result in less blood loss and pain and faster recovery times, as any incisions made are smaller and are more precise.8 Other users also report that RSS result in shorter hospital stays, less need for transfusions, and pain relievers post-operatively.10
The da Vinci RSS comprises three components; a surgeon’s console, a patient-side robotic cart with 4 arms (one to control the camera and the other three to manipulate the instruments) and a high definition 3D vision system. Da Vinci senses the hand movements of the surgeon and electronically translates them into scaled-down micro-movements so it can manipulate miniature surgical instruments. Any tremors of the surgeon’s hand movements are also easily detected and filtered out so they are not
reproduced by the unit. The beauty of da Vinci, is that the surgeon’s console is provided with a realtime stereoscopic image beamed to it from the camera built into da Vinci. Proponents assert that the advantages of RSS, are that they result in less blood loss and pain and faster recovery times, as any incisions made are smaller and are more precise.8 Other users also report that RSS result in shorter hospital stays, less need for transfusions, and pain relievers post-operatively.10
According to critics of RSS, there are a lack of studies that indicate that long term results are superior, there is often a steep learning curve, requiring additional surgical training to operate the system.11 Whether the purchase of RSS are cost effective (between $1.75-1.8M), surgeon’s opinions vary widely, mostly because some surgeons consider the learning phase too intensive, as they need to complete at least 12- 18 procedures before they comfortably adapt to the RSS.8 During the training phase, some surgeons suggest that MIS can be twice as long as traditional surgery, resulting in patients being kept under anaesthesia longer and ORs open longer. Though, based on patient surveys, RSS provide for lower morbidity outcomes.10
Regardless of the mixed opinions of surgeons, today on-site RSS have a multitude of applications which include general surgery, cardiothoracic, cardiology/ electrophysiology, gastro-intestinal (GIT) surgery, gynaecology, neurosurgery, orthopaedics, paediatrics, urology and vascular surgery.8 Many examples can be cited where RSS have set new precedents in the field of robotic surgery, such that today they have become common tools in the field. For example in 2000, da Vinci was used to perform oesophageal and pancreatic surgery for the first time in the world.12,13 Later a pancreatectomy and the first fully robotic Whipple surgery was performed. Later, in 2008, the world’s first fully MIS liver resection for a living donor transplant was performed.14 Since the first robotic cardiac procedure in the U.S. in 1999 at Ohio State University, the same group of doctors (Michler, Crestanello & Vesco) have gone on to perform coronary artery bypass graft, mitral valve, oesophagetomy, lung resection, tumour resection, and other procedures, and today their institution serves as a training site for other surgeons.8 Similarly, RSS are being used today to perform three types of heart surgery, those being; atrial septal defect repair, mitral valve repair and coronary artery bypass.15
RSS, using ‘Zeus’ or ‘da Vinci’ have been used in GIT surgery to perform colonic resection and oesophagetomy. This has been echoed in the gynaecology field, where RSS have been used to treat fibroids, abnormal periods, endometriosis, ovarian tumours, pelvic prolapse and female cancers via the transvaginal approach for a number of years. Gynaecologists now also routinely perform hysterectomies, myomectomies and lymph node biopsies using RSS. No doubt, as surgical experience and robotic technologies develop further, it is expected that the repertoire of on-site RSS procedures may expand even further.
Most Da Vinci units are located in major centres of capital cities, and it is estimated that they are commonly used in up to 450,000 operations per year globally.16 Though, while they currently dominate the RSS landscape, they are not without their problems.
Firstly, they use proprietary software, and postinstallation, each machine collects more than $100K in maintenance service agreements, plus the costs of ongoing, expensive surgical consumables.16 They are also heavy kits of machinery, weighing more
than half a tonne. This from a military point of view of course, renders them somewhat ‘immobile’ and limits their deployability.19 However, from the ‘base’ model of da Vinci, modifications have been made to develop robots with other military applications.
It should be noted that most public hospitals in Australia have not acquired the ‘da Vinci’ RSS, due to the high consumable costs of operation, and also to the belief that the evidence for their use is not strong. Having said that, there are currently 10 da Vinci machines in use in Australia, in both major public and some private hospitals, including three in Sydney and three in Melbourne, two in Brisbane, and one each in Adelaide and Perth. While there is an evident polarity regarding the usefulness of da Vinci among many surgeons, those that favour the machine are strong supporters of this technology, whom believe that the da Vinci RSS is an excellent tool that can produce amazing patient outcomes, but which ultimately requires its surgeons to be well trained and experienced, otherwise complications could be caused by the actions of the surgeons themselves.17 Also, it should be taken into account that each new version of the robot incorporates several small but significant improvements to reduce risk of patient harm.
‘Remote’ Robotic Surgical Systems
As far as remotely-applied RSS, and although they still have some way to go, they are starting to make their way into reality.16 This is the kind of technology that could provide remote surgical care in the field, and the military and private companies are investing in this idea, to make remote surgical interventions possible, thus providing a semi-autonomous technology that can provide attractive options for situations with limited access to medical care.
Envision a scenario of the future in which a “man down, man down” message comes across a military radio. Almost immediately, a casualty extraction UAV is despatched and collects the injured soldier from the battlefield and accommodates him in a mini-OR (inside the UAV itself) while flying away to a safe zone.16 An assessment and diagnosis are reached after scanning the soldier’s body for injuries, and surgery begins to control the injuries. Once those injuries are effectively treated, the soldier is evacuated via casualty extraction UAV to a base hospital.16
Although the above scenario may probably be deemed ‘too futuristic’ a system known as ‘Trauma Pod’, actually exists and it is being developed in incremental stages by DARPA.17 ‘Trauma Pod’, is a project designed to develop robotic diagnosis, life support and surgical capabilities to remotely provide medical care to soldiers injured in the field, which involves the equivalent of a futuristic operating room, in which the only human present in the room is the patient.16 The demonstration of this system, consisting of a surgical robot, robotic assistants, an integrated life support system and an intra-operative imaging system, is to perform procedures common to the battlefield, on a full-sized mannequin patient.16 The feasibility of this project has been demonstrated by the dynamic ‘choreography’ of a team of robots moving around a patient while exchanging tools and supplies.16
Interestingly, another new RSS, called ‘Raven’ has recently (2012) appeared. Originally designed for the US Army as a prototype for robotic surgery on the battlefield, this RSS, unlike da Vinci, is the first surgical robot to use open-source software (Linux- based operating system which allows modification of the original code), and in stark contrast, is compact, light and significantly cheaper ($250K).18 The Raven RSS has the disadvantage though that it is not yet approved by the US FDA for use in human surgery, so essentially, at this stage, it is still only an ‘experimental’ RSS, limited to perform operations on human cadavers and animals. It is expected though, that having put enough of these new RSS through their paces, and over time, they will overcome the hurdles of registration for human procedures. One significant dilemma that Raven will face is that its main competitor, Intuitive Surgical, holds the patents to these technologies, thereby risking the possibility of a legalistic issue in the future.
Another remote RSS, developed by SRI International consists of two lightweight 6-degrees-of-freedom arms, each weighing 4.5 kg, that can be carried in small rugged cases and quickly deployed in the field. Such systems are designed as smaller, portable, surgical systems that can function in rugged environments, such as SRI’s ‘M7’ RSS.16
Of course there are also other robotic systems, not necessarily RSS, but rather robotic ‘life support’.
One such system in this category is known as ‘Life Support for Trauma & Transport System’ (or LSTAT), which is a snake-like robotic arm attached to a high tech stretcher designed to medically attend to injured soldiers.19 This ‘snakebot’ is wirelessly controlled by a human operator with a joystick, and using its sensors and camera, it can monitor a soldiers condition. Containing a ventilator, defibrillator and other physiological monitors (oxygen saturation rate) to perform preliminary diagnostics, the stretcher attached to snakebot is basically a small, portable intensive care unit.19 The serpent-like flexibility of LSTAT allows this robot to easily manoeuvre over any point of a soldiers body, making it a useful tool to conduct an initial medical assessment in the field, being particularly useful where casualties cannot be easily evacuated when under fire. Using this system, a doctor can move the robot anywhere over a soldiers body to assess his injuries, until he can be evacuated.19 One of the drawbacks of LSTAT is that casualties still need to be loaded onto the stretcher, thereby increasing the risk to medics, but once loaded, medics can use the onboard equipment to attend to injuries. Further development is continuing to fully automate the system so that sensors move to immediately work on the casualty, while the stretcher is evacuated by UGVs. Another drawback of LSTAT, as opposed to a human operator, is the lack of tactile information. Some military trauma physicians feel that there is no evidence that robots perform better than human operators with respect to medical assessments, particularly in patients with severe trauma. While opinions vary as to the effectiveness of LSTAT, it is imperitive that such systems, at the very least, do not slow the process of diagnosis, treatment and transport, when compared to human operators. Carnegie Mellon University, who initially developed the Snakebot concept, in association with the U.S. Army’s Telemedicine and Advanced Technology Research Centre, who developed LSTAT are currently collaborating to address these issues.
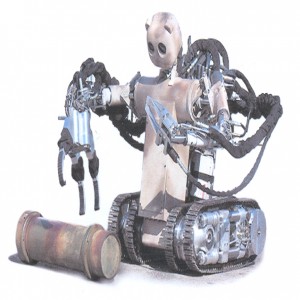
Figure 2. Battlefield Extraction Assist Robot (Source: https://www.vecna.com/innovation/bear accessed
6 August 2013)
Despite the huge strides made in the development and sophistication of RSS, particularly as they relate to both efficiency and accuracy of surgical robots, there are still many technical issues which need to be ironed out. The first relates to the delay in transmission, known as ‘latency’, (time taken between what happens at one end and what happens at the other). The second relates to the interrupted transmission of the electronic signal, known in the field as jitter, which can make the difference between a successful surgical procedure and an unsuccessful one.19 Inevitably these hurdles will be overcome. Ultimately, the concept of remotely controlled medical care is moving toward one of human-supervised autonomous operations, in which robotic devices are capable of interpreting and acting on sensor data to provide better feedback to the surgeon.16 However, mostly due to bandwidth limitations, it is likely that semi-autonomous or ‘supervised’ procedures may enter this field much quicker than remotely- controlled RSS. Having said that, humans will always remain behind the decision-making process.
Classification of Ground-based Unmanned Vehicles and Platforms
UGVs are by definition, UVs that operate on the ground, however when armed, they are commonly referred to as unmanned weapons systems (UWS). Under the UV category, though in a class of their own, also under the RGPs, which are either quadrupedic or bipedic ‘robots’, and unlike their UGV ‘cousins’ they use robotic limbs, rather than a wheel or tracked-chassis, to achieve movement. In general, UGVs and RGPs were designed specifically for dangerous missions, where a human operator could not be used. Similarly to UAVs, UGVs generally have onboard sensors to scan and monitor their environment, usually achieved either completely autonomously or via a human ‘controller’ located in another location. This distinction provides the two main categories under which UGVs and RGPs generally operate, those being; remotely-operated and autonomous.
Battlefield Casualty Extraction Robots
An important semi-automonous (remote) RGP, designed to locate, lift (scoop) and rescue people out of harm’s way, is the ‘Battlefield Extraction Assist Robot’ or BEAR (Figure 2). Developed by Vecna Technologies of Cambridge MA, and funded by the US Army Medical Research and Material Command, BEAR was designed as a powerful, highly agile, mobile robot.20,21 Standing at 6ft high when extended, its upper torso has two arm actuators which are extremely strong, whereas its lower body base consists of highly manoeuvrable tank tracks, which can separate out as thighs and calves, giving BEAR extra height when required.20,21
Designed to negotiate rough and uneven terrain, in its kneeling position, it travels over rubble using its tracked ‘legs’. The robot’s sense of balance and coordination are controlled gyroscopically using Dynamic Balance Behaviour technology and computer-driven motors which enable it to stand and carry loads upright on its ankles, knees or hips for up to an hour at a time.21 Interestingly, it can even negotiate stairs while carrying a wounded soldier, as well make its way through most standard doorways. With a top speed of approximately 10 km/hr, and a hydraulic upper body having the capability to lift up to 227kg (500lb), this robot is very well placed to carry out it’s core mission of casualty extraction.20,21
BEAR ‘sees’ via use of its inbuilt cameras, and ‘hears’ via use of its inbuilt microphones. While the early prototype (vers. 6) essentially can carry out all of the above functions, the latest model (vers.7) has undergone a number of improvements. One of the most significant of these has been to give BEAR a ‘friendly’ face, which its designers felt was important, so as to re-assure casualties and allay their fears. Other design modifications include a stronger and sleeker, ‘humanoid’ upper torso, Actin software integration (from NASA) for controlling and coordinating limb movement, ‘finger-like’ end effectors, and inbuilt detectors for chemical, biological and explosive agents, using Laser-Induced Breakdown Spectroscopy.20 The latest efforts include implanting pressure sensors in the effectors to ensure that human casualties are handled with ‘sensitivity’. One can easily deduce that this RGP would also have useful applications in the civilian area of emergency medicine, such as the retrieval of victims from hazardous road accident environments, from damaged buildings after an earthquake, or simply to move immobile patients in a hospital.
Another robotic casualty extraction system to come onto the military market recently is the ‘First Responder Robot’ from Hstar cRONA. The beauty of this RGP is that it provides the ‘traditional’ functionality of mobility, telepresence and casualty lifting capabilities, but also diagnostic capabilities including ‘in-field’ ultrasound.22 According to its developers, future upgrades will include autonomous ultrasound image acquisition, 3D ultrasound imaging and visualization, infra red scanning and autonomous traumatic injury assessment and desired treatments will be possible via a medic operating the system remotely.22 Of course, ‘First Responder’ has, similarly to BEAR, the same capabilities to operate in hazardous conditions including fire, biological, chemical and even radioactive environments.
Load-Carrying or ‘Porter’ UGVs and RGPs
In recent years the task of carrying logistic loads (medical supplies; munitions; weapons) has become an ‘automated’ function, and lately it has been greatly enhanced by the array of ‘porter’ UGVs and RGPs, which have become available. Not only having the ability to carry heavy loads (227kg) over long distances and over rough terrain, but also to act as ‘escorts’ to accompany small squads (3-10) soldiers on both operational and logistical missions.23,24 This they can do quietly, for up to 72hrs without refueling, and one example is the ‘REX’ porter UGV (Figure 3).23
Essentially, the REX UGV follows the soldier or medic that operates it remotely. Alternatively REX can be programmed to trail soldiers up to 6 metres away, via use of a small remote control device.23 The functionality of these systems has not only enhanced the performance of infantry combat units in the field (as soldiers can carry more supplies to accomplish their mission) but it has also enhanced the ‘mobility’ of field medical missions, particularly where the REX UGV has an RSS on board. In terms of operability, the REX UGV is hard-wired to move at the same pace as that of the soldiers or medics on patrol, to come to a stop when required, and to either reduce or increase its operating speed.23,24
Currently, a series of UGVs and RGPs are in development, each being designed with a function in mind. For example, REX, and other similar prototypes (designed to accompany combat infantry units) are being armed with an array of lethal weapons, an example of one of these being the ‘CaMEL’ UGV.25 The core mission of these armed UGVs is to serve two purposes, to manoeuvre with small units and conduct ISR, and secondly, to close in on and destroy the enemy.25 Interestingly, the CaMEL UGV can carry 200kg of supplies over a 72 hr mission, while maintaining a 4km/hr steady march speed for 8 hrs, and with the ability to jump from zero to 38 km/hr bursts for up to 200 metres, and on slopes between 30-60 degrees.25
Some UGV and RGP models are being designed exclusively for ISR whereas others are purely as medical logistics and treatment platforms. Aside from military applications, the civilian ‘Remote Package Handling System’ (or RPHS) from the Provectus company, has been exclusively designed to operate at airports where its core function is to assess and quickly remove ‘suspect’ packages from airport terminal buildings, thus reducing risk to airport employees, as well as minimizing both loss of revenue and downtime.26
An innovative autonomous RGP is the US Army’s ‘Big Dog’ (Figure 4),27 which is a robotic quadruped, designed to carry equipment for ground troops and medics over difficult or rough terrain. Also known in the US Army as the ‘Multifunctional Utility/Logistics and Equipment’ robot or ‘MULE’. Weighing 110kgs and standing 0.76m tall, it can carry 154kgs at an average speed of 6km/h, and climb hills at an incline of up to 35 degrees.27 Big Dog has the capability to jump over low obstructions, climb over low vertical obstacles, walk on ice and importantly, it never falls off its feet.27
Current limitations of UGVs and RGPs
While some of the autonomous functions of UGVs are well advanced (such as mobility, endurance, communications and navigation), the development of behavioural functions relating to their adaptability and employment in complex tactical scenarios is still at an early stage. One particular issue is whether to limit UGVs (and other robotic technologies) to adaptive control solutions or whether to incorporate artificial intelligence, ultimately seeking UGVs capable of complete and ‘responsible’ autonomous operation.
What are the advantages of RSS and UVs for the ADF?
Undoubtedly, the most valuable advantages of RSS is their ability to perform critical life-saving surgical interventions and physiological monitoring, whereas UVs can facilitate combat casualty extraction and evacuation. UVs can also do ISR tasks, and aid and complement the mobility of medics and doctors on the battlefield, while at the same time, protect the operator from direct fire using their own self-defence mechanisms. Such features have made both RSS and UVs attractive to armed forces and law-enforcement agencies alike, including unconventional warfare and counter-terrorism operations.
UGVs and RGPs are versatile, agile and relatively rugged. Moreover, with the ability to perform repetitive tasks with speed and precision—and being devoid of human emotion—they are tenacious, tireless and fearless. This makes them extremely useful for a range of mundane, tedious and dangerous tasks on the modern battlefield, especially ones that would otherwise expose human operators to higher-than- normal risk of injury or death. Similarly today, with the rapid pace of research and development in this area, it is hoped that small portable RSS will be able to provide the capability of remote surgical interventions, as well as advanced life support in the field, in the near future.
Moreover, as the development and proliferation of RSS, UGVs and RGPs continues, their acquisition costs will reduce, making them more affordable for militaries around the world, particularly where their employment can reduce overall manpower requirements or, it is hoped, minimise the risk of death or injury to service personnel. These attributes have been recognised by the US Congress, which mandated in 2000 that one in every three future US combat systems should be unmanned.28
For the ADF, the potential utility of these technologies—and ultimately their effectiveness and reliability on the future battlefield—will need to be weighed against specific mission requirements and detailed cost benefit analyses. However, the drawbacks of these technologies which include significant expense, the question of how to provide an ongoing power supply for prolonged missions, and reliable evidence for the efficacy of these technologies all need to be considered. On the one hand, it is relatively easy to justify the acquisition of a particular UV to meet a specific, existing capability. The considerably more difficult exercise is to contemplate the required force structure for a future battlefield involving a combination of manned and unmanned platforms and systems, operating as an integrated combat and medical battlefield network.
The other challenge, which has been addressed by a number of commentators— in earlier issues of the Australia Defence Force Journal as well as other scholarly publications—is the complex question of the ethical, legal and political implications of employing increasingly autonomous robotic technologies in operations.29,30,31 While some might argue that issue is overblown and the stuff of science fiction novels, it seems inevitable that in the long term future, UGVs and RGPs will progressively incorporate artificial intelligence systems, giving them increasing levels of autonomy, but not complete autonomy from the human operator. Remote RSS however (unlike on-site RSS) are still at an experimental stage of development, hence they may provide potential future advantages in the field, though these are currently only ‘experimental’ at best.
Conclusion
While the possibility of using robotics on the battlefield to conduct warfare operations has long been envisaged by military planners, it has not until recently been recognised that these very same technologies can also be developed to enhance the practice of battlefield medicine and trauma care. It seems certain that RSS, UGVs and RGPs will continue to proliferate in ground medical operations, where they have the potential to greatly improve the life-saving effectiveness of medical interventions and casualty extraction, thereby reducing human fatalities on the battlefield.
In the longer-term, it seems inevitable that the battlefield of the future will be dominated by increasingly-autonomous UWS and platforms, operating across the environments of air, sea, land and space. It is now also evident that such technologies designed and purpose-built for medical applications, will also dominate the battlefield of the future. How those platforms and systems are integrated into future force structures—including for the Australian Defence Force—is a complex issue, requiring considerable analysis and planning.
This article has provided some vision of what the future battlefield medicine and associated logistics, potentially holds. Aside from RSS, in some ways, UGVs and RGPs are perhaps the ‘perfect orderly’ in the sense that they are mission-driven, highly- survivable, easily-repairable and, if required, disposable. Their effectiveness will only be enhanced further when questions regarding the human-robot interface are solved, as will be their repertoire of uses within the military medical organisation, as increasing levels of operating autonomy are achieved.32,33 It seems that, as many futurists will argue, the ‘age of the machines’ has truly arrived.
About the Author
In his role as Laboratory Manager and Supervising Scientist, the author manages the Centres for Transplant & Renal Research and Infectious Diseases & Microbiology, at the Westmead Millennium Institute for Medical Research (Westmead Hospital). He is also the Training Officer-Operations and an Instructor with the Australian Air Force Cadets (AAFC), based at 307 (City of Bankstown) Squadron, located at AAFC Headquarters, Lidcombe Multi-User Defence Facility, NSW. With a lifelong interest in military aviation and military history, and having served for a number of years in the Air Training Corps, as a serving AAFC Officer, he now dedicates a significant portion of his time to the supervision, training and mentoring of AAFC cadets. He has a strong interest in unmanned and future weapons systems of land, sea and air.